ARCE Newsletter, No. 127 (1984) pp. 24-43
Reproduced by permission of the American Research Center in Egypt.
Introduction. My previous studies of the Sphinx concern the weathering of stone and means for arresting the decay phenomenon (Gauri, 1981, 83; Gauri and Holdren, 1981). This article delineates the geological framework as a reference for future studies.
In the course of my field work at the Sphinx, including a 5-week intensive study during November-December 1981, the necessity of conducting basic geologic work became apparent. In filling this need, Mark Lehner, Director of the Sphinx Project of the American Research Center in Egypt, was most instrumental, especially due to his keen insight into natural phenomena and his skill in the use of survey equipment. In minimal time we had a topographic map of the Sphinx area which enabled us to place the newly recognized geological horizons in their precise geographical location. This was also aided by the newly developed photogrammetric map of the Sphinx by Ulrich Kapp of the German Institute in Cairo. Mr. Lehner and I worked together in the field throughout my several visits to Giza during 1980-82. Dr. James P. Allen, then the Director of ARCE in Cairo, selflessly devoted many hours to facilitate our work and sponsored my visits to Cairo and other places to obtain a broader perspective of the conditions of monuments in Egypt. In this connection, I also wish to recognize the help of Dr. Qadri, President, Egyptian Antiquities Organization in inviting me to Cairo for a week in 1982 during which I had the opportunity to visit ancient quarries in the Mokattam Formation, east of the Nile River.
I am most gratified by all these associations and the results that have sprung from these, i.e., the realization among all concerned that scientific investigation is essential to our understanding, particularly of the phenomenon of stone decay and for guidance in selecting appropriate techniques of conservation.
Regional Setting and the Materials of Study. Located at the southeastern flank of the Giza Plateau, the Sphinx is carved from the middle Eocene limestone of the upper Mokattam Formation.
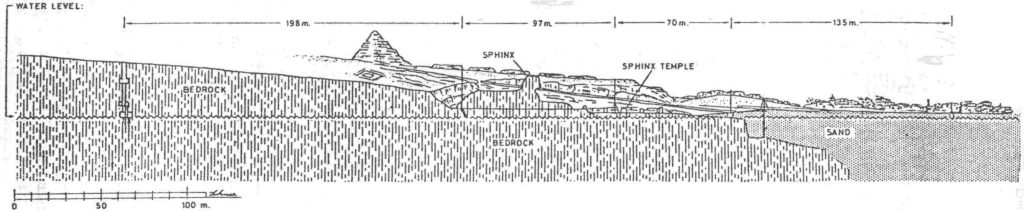
Due to the southeasterly dip of the strata and the low elevation (appx. 100m.) of this limestone plateau, the equivalent of rocks occurring in the head region of the Sphinx have not been found elsewhere to the immediate north, east, or west. They are even absent in the southerly direction due to extensive quarrying marking the site of the Pharaonic Necropolis. However, the rocks of the thoracical region of the Sphinx are widespread in the Pharaonic Necropolis. Farther south, these rocks disappear under the next younger Maadi Formation and the desert sand. The following study therefore is based on rock samples from the core of the Sphinx and the walls of the ditch surrounding it in all directions except in the east where the Sphinx sanctuary is located. Large limestone blocks used for enclosing and partitioning the sanctuary appear to have been extracted from the site of the ditch (Lehner, 1980; Aigner, 1982).
The lower portion of the Sphinx is veneered with dimensional limestone blocks. Some of these blocks, used during several restorations beginning with the pharaonic times, were extracted from a variety of geological horizons from quarries located in the Eocene beds right of the Nile River. Since the stones of the Pharaonic restoration have not deteriorated at all over these nearly 5,000 years of exposure at the Sphinx, we have studied the porosometric properties of these stones with a view to determine criteria for selection of durable stones for future restoration (Gauri, 1981 and 1983).
Stratigraphy and Sedimentology of Rocks at the Sphinx. The limestones exposed at the top of the Giza Plateau as well as those exposed at its bordering cliffs have been assigned to the Mokattam Formation (Said, 1962). The last derives its name from the Mokattam Hill at Cairo, east of the Nile River. The literature review reveals a general consensus of opinion that there is a good stratigraphic match between the limestones on both sides of the Nile at Cairo. However, the portion of the Mokattam Formation studied in the Sphinx area shows, in itself, a distinct sedimentologic development which allows us to divide this portion of the Mokattam Formation into three members (Plate 1, Fig. 2, and Fig. 3.) The proposed names of these members are:
- Akhet Member
- Setepet Member
- Rosetau Member
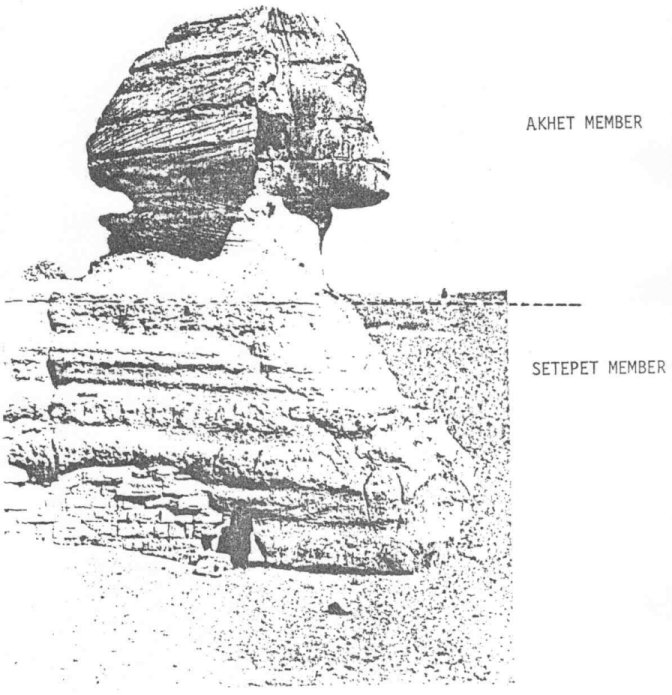
* These names were suggested by Mr. Lehner
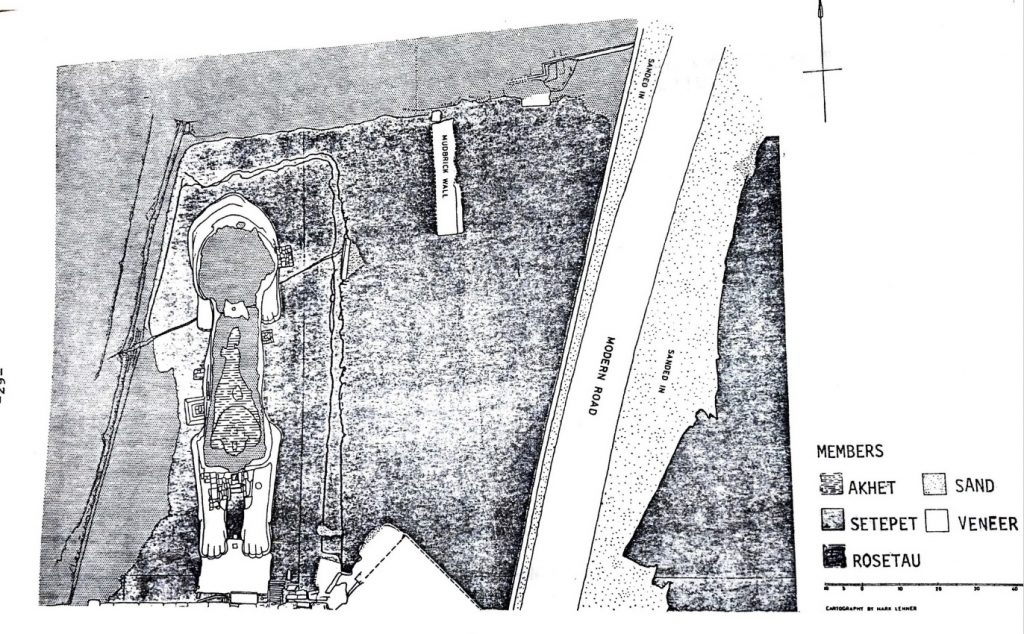
Rosetau Member (this name derives from the ancient Egyptian place-name of the Sphinx precinct in the 18th Dynasty). The lowest member in this area, it is a massive reefal (bioherm) limestone with a knobby surface having a relief of nearly one meter.
This member is well exposed in the wall of the north ditch whence it continues and forms the upper surface of the slope leading to the modern road to the pyramids. It also forms the lower part of the western and the southern wall of the ditch. At the core of the Sphinx, this member is largely covered beneath the veneer stone. However, it outcrops at the floor of the south ditch. In the south-east corner of the south ditch, this horizon dips under the Setepet Member.
Setepet Member (the name is derived from ancient Egyptian STPT, the New Kingdom name for the immediate Sphinx sanctuary). The Setepet Member is nearly 10 meters thick sequence of six parallel bedded layers each ranging in thickness from 1 to 2 meters (Fig. 3A).
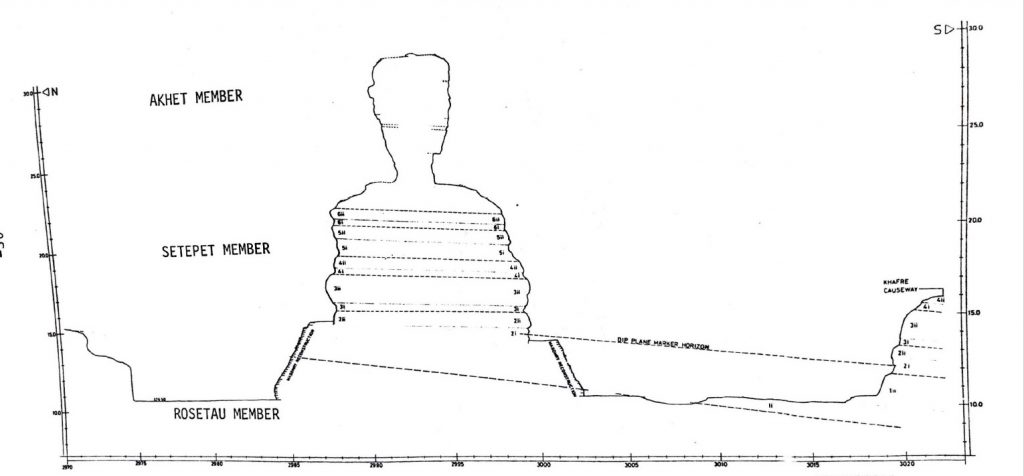
Except layer 5, all other beds become progressively thinner towards the upper reaches of this member. The differential weathering has prepared this sequence into distinctly alternating projected and recessed layers.
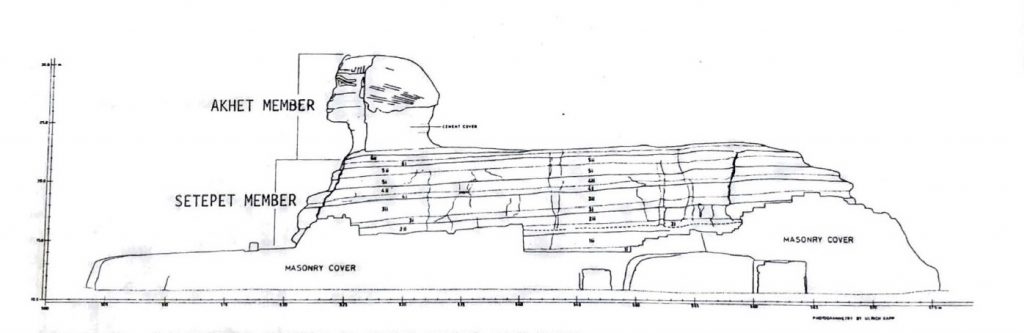
The Setepet Member forms the main core body of the Sphinx. In the south and west of the Sphinx, the Causeway and the Pharaonic Necropolis are located in these beds.
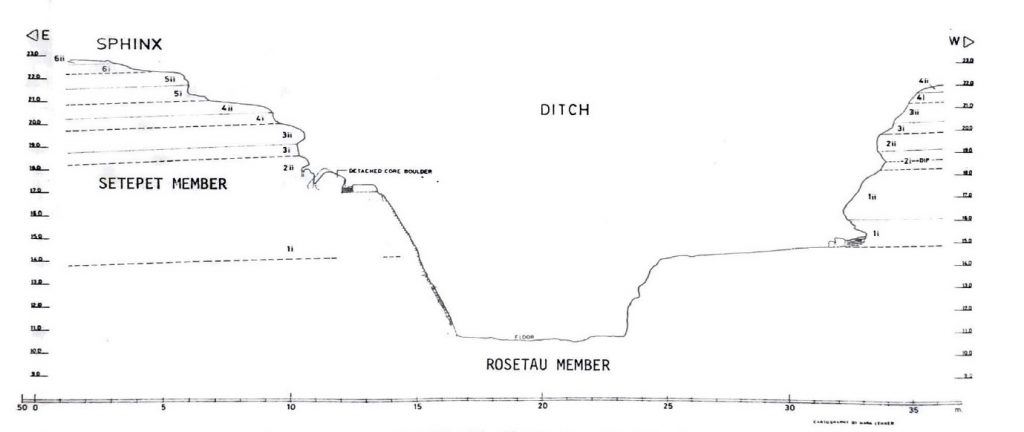
These beds reveal cyclothemic pattern of sedimentation. At the base, the hummocky topography of the Rosetau Member is largely obliterated due to the filling in of deeper portions by the argillaceous silty limestone material of the lower portion of Bed 1. By the termination of deposition of this bed the sedimentation surface appears to have been rendered flat so that a horizon in the lower portion of Bed 2 has a planar surface extending over the entire area of study. We have used this “Dip plane marker Horizon” to determine the regional dip of the strata in the study area.
The pattern of sedimentation of the Setepet Member is that from below upwards, within a single bed as well as from the base of the member to its top, there is a progressive reduction of the elastic material and the evaporite minerals such as halite and gypsum in the carbonate sediment. As a result the lower marly portion of the first bed is darker than the upper more calcareous portion. This distinction disappears upwards so that the upper beds appear to be uniformly white throughout its thickness.
The progressive reduction in the influx of the land derived clastic fraction of the sediment is indicative of the increasing calmness of the sea within the period of deposition of a bed as well as the deposition of this entire member. This genetic control of sedimentation and the change of this rhythmic activity towards the end of the deposition of this member are the reasons for our separation of this member from the following Akhet Member.
Akhet Member (ancient Egyptian term for the “Horizon of Khufu”; one of the names of the Great Pyramid and the Giza Plateau). The Akhet Member is exposed only in the neck and the cephalic region of the Sphinx. It has been either quarried away from the rest of the area under consideration or is buried under the sands in its southerly extension.
The Akhet Member is nearly nine-meter-thick sequence the lower one-third of which, forming mainly the neck of the Sphinx, is a relatively softer limestone being richer in the clastic fraction. The upper portion is a massive limestone interlayered with four distinct partings, each nearly 10cm thick, of somewhat softer limestone similar in composition to the limestone of the neck.
The depositional history of the Akhet Member is similar to that of the Setepet Member. In the Akhet Member, however, only two distinct depositional episodes are discernable namely, the deposition of the lower marly portion during conditions of somewhat turbid water and the sedimentation of the cleaner carbonate of the upper portion during conditions of calm water. Small quantities of gypsum were also precipitated from the water and were incorporated in the calcareous sediment. This gypsum presently forms the duricrust which gives the brownish appearance to the head region and has contributed towards its durability.
Petrography of the Rocks at the Sphinx. The rocks of the Sphinx are a series of limestone beds with their characteristic large foraminifera, the Nummulite gizehensis. These limestones, studied under the petrographic microscope are extremely fine-grained packed biomicrite (Blatt et. al. 1972) in which the skeletal remains are mainly that of foraminifera embedded in a small amount of carbonate mud. The sparry calcite occupies the cavities in fossil shells.
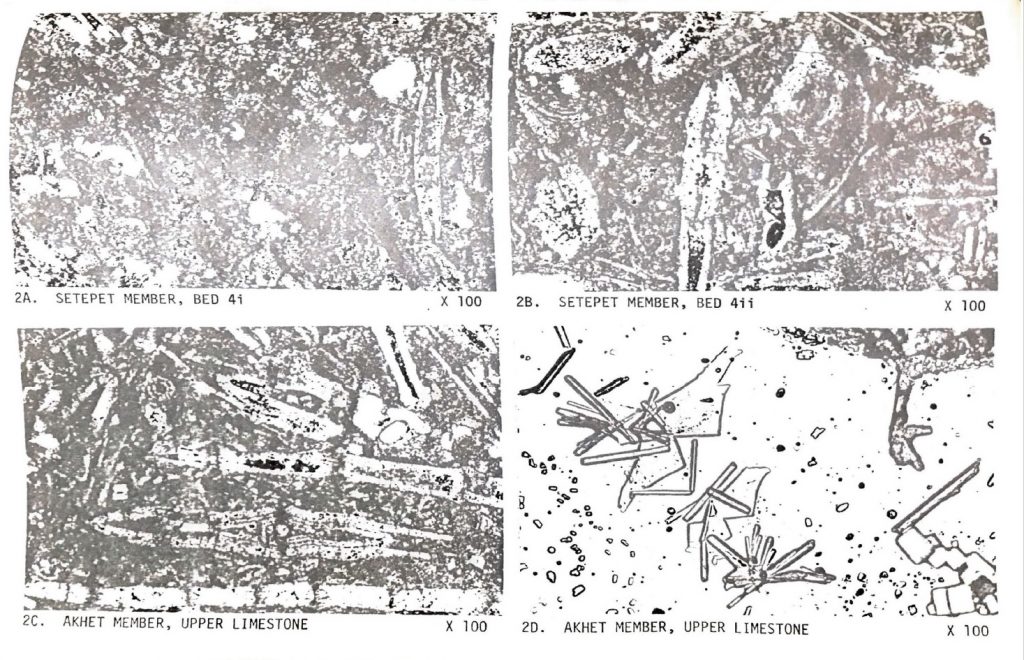
Figure 2B Setepet Member, Bed 4ii (x 100)
Figure 2C Akhet Member, Upper Limestone (x 100)
Figure 2D Akhet Member, Upper Limestone (x 100)
Under the useful magnification achieved by the petrographic microscope, the difference between the limestones of the Sphinx is based on the variation in the size of the allochem, i.e., the skeletal grains. The limestone specimens of the upper portions of each bed of Setepet Member and those from the upper Akhet Member have much larger tests than the specimens from the marly lower portions of these formations. Also the grains of silt and hematite are far more abundant in the later specimens. As a result, the petrographic thin sections of the lower portion of a bed are browner than those of its upper, and the brownishness is successively lesser in the upward direction of Setepet Member.
These variations in properties, i.e., the grain size, etc. do not significantly control the behavior of the limestone towards weathering. But it is readily apparent from Plate 1 that the rock units have undergone differential weathering. For a complete characterization of the limestone, therefore it was essential to segregate the components of the limestone in order to resolve those which are present in extremely small quantities. For instance, the water-soluble salts were extracted by placing powdered limestone in deionized water. This water then was analyzed in two ways:
1. directly by atomic absorption spectrophotometry and by wet chemical techniques for quantitative analysis of the ionic composition (Table 1).
2. the salts were precipitated from this water and then studied under a petrographic microscope (Pl. 2) and x-ray diffraction (Fig. 4) to determine the mineralogical composition.
Further, for the purpose of concentration of other possible minerals in the limestone relative to calcite, the samples were powdered to approximately 300 mesh and digested in 10% acetic acid for two hours. The fine residue, while in a state of suspension, was collected on filter papers and analyzed by x-ray diffraction. The following are the results of these analyses:
Water Soluble Salts. Two major water soluble salts have been identified as gypsum (CaSO4 .2H20) and halite (NaCl). A less stable phase of calcium sulfite, namely 2CaS04.H20, has also been identified in the precipitates, but it cannot be determined whether this phase occurs in the rock as such or that its existence in the precipitate is due to the chemical controls relating to ionic concentrations, temperature, and rate of crystallization, etc. Gypsum and halite are present in all the studied samples. Plate 2 shows the crystal of these salts growing from the stone extract in deionized water on petrographic slide. Fig. 4 shows these salts as identified by x-ray diffraction. The ionic compositions of these salts are given in Table 1.
The relative abundance of the salts is of great interest. Gauri and Holdren (1981) reported that halite is present in larger quantities in the lower portion of each bed of the Setepet and Akhet Members while gypsum occurred in larger quantities than halite in the upper portion of each bed. Table 1 reveals some variation from this, even though a similar pattern is easily discernable. The variation is probably due to sampling: the specimens for 1981 study were derived from the weathered surface of the Sphinx core while the present samples are mostly from the unweathered portion of respective rock units.
Non-Carbonate Clastic Fraction. The major properties of the fraction consisting of clay minerals is revealed by the x-ray diffraction spectra. The 20, 5-15% reflections indicate a large d-spacings in the range of 5 to 15A which are characteristic of clay minerals. The identification of individual clay minerals is permitted by the following data.
Table 1. Water soluble salts in the lower four beds of Setepet Member; expressed as weight percent of stone: The designation i in each bed refers to the lower marly portion of the bed and ii is for the upper more calcareous portion.

Table 2. Carbonate and clastic materials in the lower four beds of the Setepet Member; expressed as weight percent of stone.


The kaolinite occurs in all the studied samples but Illite and Montmorillonite have been identified in the upper limestone of the Akhet Member and in Bed 3 of the Setepet Member.
The percentage of the non-clastic fraction follows a definite pattern (Table 2). The lower marly portions of the Setepet beds have larger quantities of these, relative to the respective upper limestones. The Akhet Member follows the same pattern.
Carbonate Fraction. Needless to say that the most abundant mineral species is calcite; aragonite or magnesium carbonate has not been identified. The nature of the calcitic constituents has been discussed earlier in somewhat greater detail.
Structural Geology of the Sphinx Rocks (K.L. Gauri and D. Dockstader*). The Sphinx region is a part or the Egyptian unstable shelf of which the structural features are minor folds and extensive fractures and faults. At the Sphinx, joints criss-cross the bedrock. Joint orientation was measured in the rocks at the Sphinx and those forming the walls of the Sphinx Ditch. A structural map of the Giza Pyramid region is reproduced from Omara, 1952 for a large perspective of the joint patterns.
The regional attitude of the bedding along the Nile Valley is a gentle northward dip so that the strata are progressively younger from Aswan towards Cairo. At Cairo however the strata dip towards the south indicating towards the presence of a local anticline termed as Brachy-Anticline by Omara. Precise mapping (Fig. 3A) of strata exposed at the Sphinx core and the walls enclosing the Sphinx Ditch shows that the dip of the plane of contact between the Rosetau Member and the Setepet Member is larger than the dip of the following strata and that the beds become progressively gentler towards the top of the sequence. This indicates towards the occurrence of the southward dipping paleoslope prior to the deposition of these upper Mokattam Strata. This inference is supported by the following analysis of the joint patterns.
Joint orientation were measured and since the attitudes appear consistent in each of the three members, the data has been combined in a single diagram. This diagram, shown in Figure 5 is a density contour plotting of poles to 84 joints. This figure shows that the joints for the most part fail into two orthogonal groups. One of these groups trends generally northeast to southwest while the other trends northwest to southeast. The centers of these clusters are precisely perpendicular, with trends of N36W and N54E. This general pattern is also seen, though less conspicuously, in the map reproduced from Omara.
The observed orthogonal joint pattern is characteristic of extensional regimes resulting from mild deformation. Similar patterns are frequently observed over gentle anticlines or domes. However, these strata show no evidence of the deformation usually associated with the joint distributions observed. The explanation for these joint patterns apparently lies in the underlying Cretaceous sediments. These rocks have been bent into a series of anticlines, with a northeastern trend and also contain fractures and faults in a NW-SE direction as well as parallel to the fold axes. These features are the result of late Cretaceous tectonic activity (Salem, 1976 and Said, 1962).
Since joints in the undeformed Eocene strata have the trends of fractures in the Cretaceous units, it seems likely that the regional post Eocene epeirogenic movement, perhaps associated with the opening of the Red Sea and the Gulf of Suez, has caused the Cretaceous fractures to propagate into the overlying Eocene sediments. From the intensity of the Cretaceous deformation a broader distribution of dips would be anticipated for the joints in the Cretaceous units than the distribution that has been observed in the Eocene strata. The subvertical joints in the Cretaceous units have propagated into the overlying strata more effectively than those with lesser dips. This preference is consistent with the hypothesis of propagation in an epeirogenic environment. In this setting there is no strong horizontal compression to reactivate fractures with shallow dips, and so movements would all be nearly vertical.
The existence of two orthogonal, subvertical joint groups in the rocks of the Sphinx has been detrimental to its durability. Where joint intersections occur near the edge of the Sphinx, they separate wedge-shaped blocks from the rest of the sculpture, causing massive loss of material from the core.
*I recognize the assistance of my colleague, Dr. Dockstader, in preparing this section of the paper.
K. L. Gauri
University of Louisville
Literature References
Aigner, Th. Zur Geologie und Geoarchäologie des Pyramidenplateaus von Giza, Ägypte. Natur und Museum, 112 (12), p. 377-388, Frankfurt A.M. 1982
Blatt, H., Middleton, G., and Murray, R. Origins of Sedimentary Rocks. Prentice Hall, Inc., 634 p. (p. 472: Folks classification of carbonate rocks), 1972
Gauri, K.L. The Deterioration of Ancient Stone Structures in Egypt. in Prospection et sauvegarde des antiquités égyptiennes de l’Egypte. Institut français d’archéologie orientale du Caire, N.C. Grimal ed. p. 13-18, 1981
Weathering in Deserts as Exemplified by the Great Sphinx at Gizeh. Geol. Soc. Am. Abstracts with Programs, p. 457, 1981
Removal of Water Soluble Salts from Masonry and Porosimetry of a Pharaonic Veneer Stone from the Sphinx. American Research Center in Egypt, Newsletter 124, p. 19-27, 1983
– and Holdren, G.C. Deterioration of the Stone of the Great Sphinx. American Research Center in Egypt, Newsletter 114, p. 35-47, 1981
Lehner, M. The ARCE Sphinx Project. A Preliminary Report. American Research Center in Egypt, Newsletter 112, p. 3-33, 1980
Said, R. The Geology of Egypt. Elsevier Publishing Co., 377 p., Amsterdam-New York, 1962
Salem, R. Evolution of Eocene-Miocene Sedimentation Patterns in Parts of Northern Egypt. Am. Assoc. Petroleum Geologist, Bull. 60, 1 p. 34-64, 1976